Since we aimed for simultaneous on-line measurement of multiple components ranging from highly volatile VOC (CFCs, isoprene, chloromethane, etc.) to adsorbable VOC (bromoform, chloroiodomethane, diiodomethane, etc.), we employed a silicon membrane tube type vapor-liquid equilibrium method.
Measurement method
To measure alternating halocarbon partial pressures in air and surface seawater, we applied automated air concentrator-gas chromatograph-mass spectrometer (GC-MS) technology. This is based on the National Institute for Environmental Studies' system for unattended monitoring of atmospheric VOCs on remote islands (Yokouchi et al., 2006). A custom-made automatic air concentrator manufactured by Taiyo-keishoku-sha was used and linked to a GC-MS (Agilent 5973/6890). First, seawater was constantly pumped from the bottom of the research vessel for surface seawater samples ( seawater for research). To continuously extract VOC dissolved in seawater, a silicon membrane tube vapor-liquid equilibrator was developed (Ooki and Yokouchi, 2008). An overview of the entire system is shown in Figure 1, and the structure of the vapor-liquid equilibrator is described below. Six silicone tubes (Fuji Systems, material Q7-4780, 80SH), each 2 mm in diameter and 10 m long, were bundled together and passed through a polyvinyl chloride (PV) pipe. Pure air flowed through the silicon tubes, and sample seawater flowed constantly outside the silicon tubes (inside the PV pipes). The pressure inside the silicon tube was maintained at 1.4 atm and the air velocity at 25 mL min-1. Since the pressure value is proportional to the partial pressure, highly accurate pressure control is necessary. In this system, a piezo-valve type pressure controller (Horiba Estec, PV-1000) was used. The reason for setting the pressure higher than atmospheric pressure is to ensure high-precision pressure control and to prevent seawater from entering the analyzer side through the silicon tube in case the silicon tube is damaged.
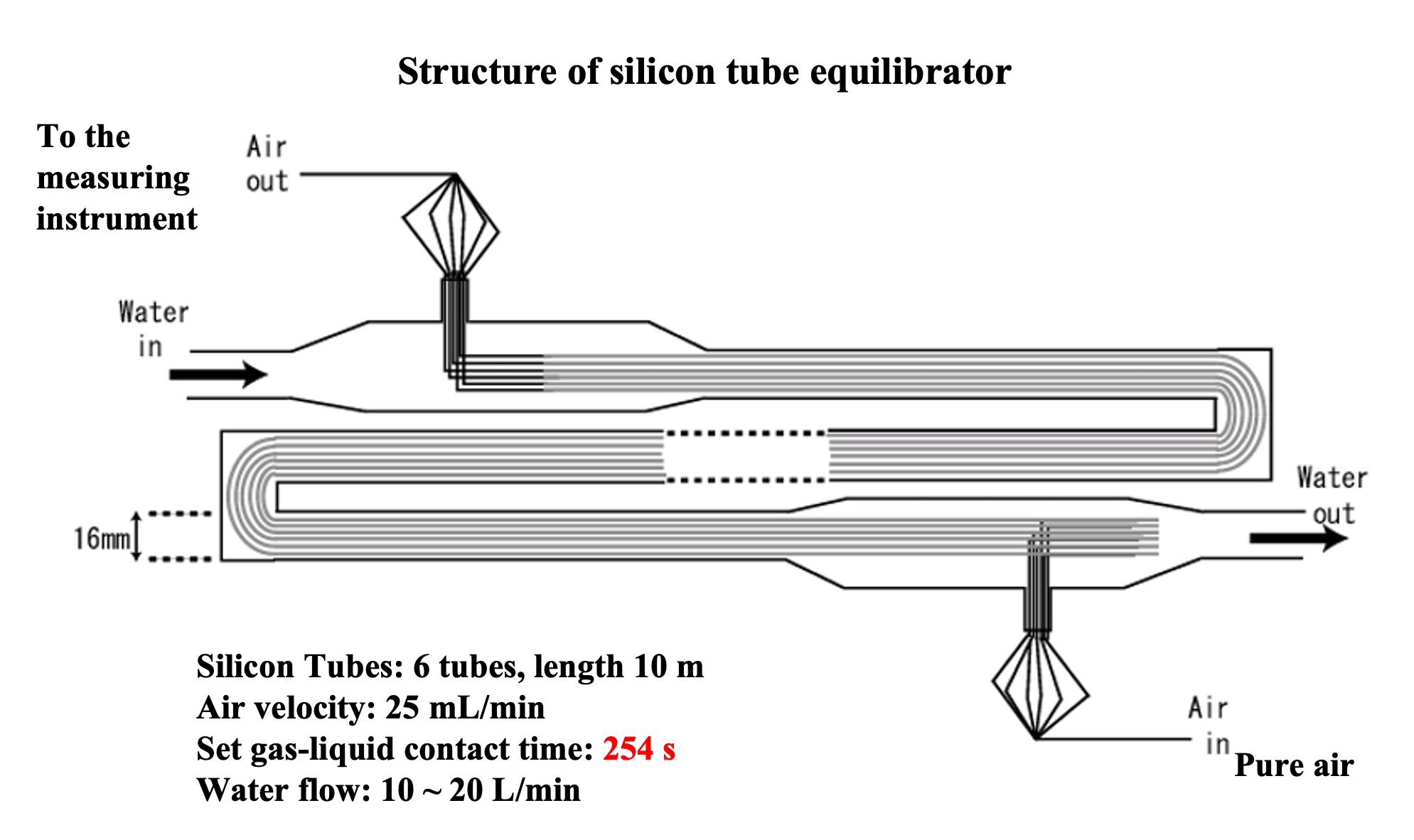
VOC in the sample seawater permeate the silicon and move to the air side of the tube. If the contact time between the silicon tube and seawater is long enough, the VOC partial pressure in the seawater and air will reach equilibrium (a confirmation test of the equilibrium state is described in the next section). The sample air was then introduced into an automatic air concentrator to cryo-focus the VOCs in two steps, and halocarbons were detected by GC-MS. In addition, outdoor air was suctioned continuously to the onboard laboratory for atmospheric samples. Since the air suction line extends several tens of meters, it is necessary to minimize the adsorption loss of VOC in the air line. Therefore, the sample was once aspirated by a high-flow pump (30 L/min) and introduced into a metal bellows pump by splitting the atmospheric line just before the analyzer. The metal bellows pump constantly introduced the atmospheric sample into the atmospheric concentrator while suctioning and pressurizing it. Halocarbons in the atmospheric sample were detected by the GC-MS system in the same way. Six sample lines were connected to the air concentrator: a vapor-liquid equilibrator line, an air line, two standard sample air lines, and a helium line (blank measurement), which could be switched by computer control. VOC in mixed VOC standard air (100 pptv halocarbons - 10 ppb isoprene) were measured in the same manner to quantify the partial pressure of VOC in the sample air introduced into the air concentrator. VOC components (CH2ClI, C2H5I) not included in the mixed VOC standard air were determined from the response ratio of CH2ClI and C2H5I to C2Cl4 by heating and vaporizing a mixed standard solution of CH2ClI, C2H5I and C2Cl4 stock solution diluted in methanol and measuring the same. The quantitative components were chloromethane (CH3Cl), bromomethane (CH3Br), iodomethane (CH3I), dichloromethane (CH2Cl2), dibromomethane (CH2Br2), trichloromethane (CHCl3), tribromomethane (CHBr3), chloriodomethane (CH2ClI), iodoethane (C2H5I), CFC-11 (CCl3F), and HCFC-22 (CHClF2).
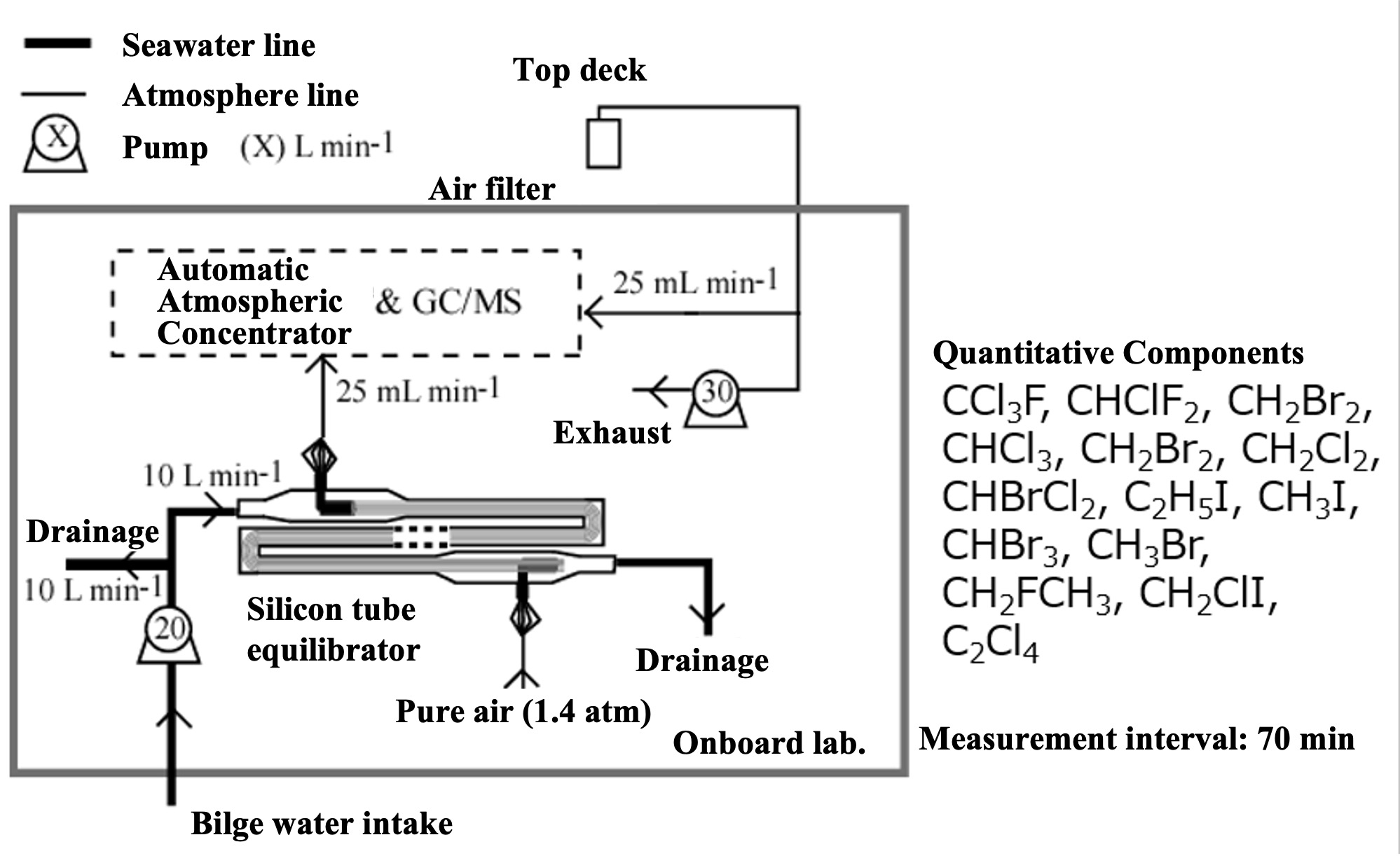